5G NR and NG-RAN
Figure 1.4 shows the end-to-end architecture for the 5G system.
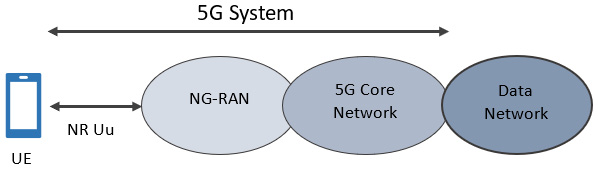
Figure 1.4 – 5G system high-level architecture
5G systems largely comprise the 5G NR, NG-RAN, and, finally, 5GC.
NG-RAN architecture
Figure 1.5 shows key elements within the architecture of the NG-RAN. The User Equipment (UE) can be in the form of a mobile device, but it could also be in all manner of different forms, as can be appreciated with the advent of IoT. In order to provide RAN coverage, there are gNBs, which stands for New Radio Node B. A radio interface is needed to create connectivity between the UE and the gNB. That radio interface is called 5G Uu. Notice also that there is connectivity between the gNBs. So, the Xn reference points allow these gNBs to communicate with each other. Finally, connectivity between gNBs and the core network is needed, which is also achieved by the N reference points. In the diagram, the N2 reference point is providing the control plane flow, whereas the N3 reference point is used for the user plane, which carries user traffic and user data.
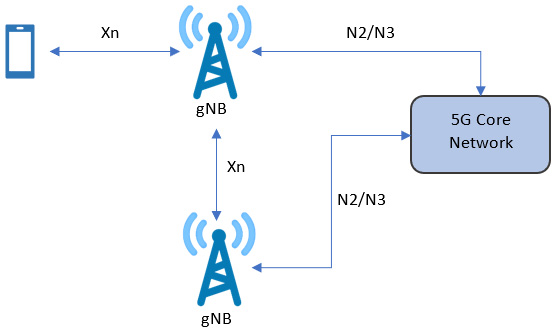
Figure 1.5 – NG-RAN architecture
The gNB is responsible for radio resource management and a big part of that is scheduling uplink and downlink data onto the radio interface. The gNB handles numerous different devices and its cell or even cells. A single gNB may be in control of several different cells. It is responsible for scheduling user data correctly onto the downlink or telling the UE when to transmit data on the uplink. The gNBs are also responsible for handovers. The Xn reference point that sits between the gNBs allows them to coordinate handovers between themselves. Security is a key factor as well, so the gNB will be involved in the security across the radio link as well as the UE. Finally, the gNBs are responsible for dual connectivity, which will be examined later in this chapter.
The UE is responsible for bidirectional data transfer via the 5G NR, ensuring a high quality of service. So, the UE needs to be sure that the correct traffic is sent to the correct bearer. By being the other end of the radio connection, the device is also responsible for security. Finally, the UE needs to support dual connectivity if it is being used in the network architecture.
Tracking areas
Looking at the NG-RAN at a higher level, we can see that there are thousands of gNBs deployed to provide that RAN coverage. In any mobile communication system, the UE is not constantly connected to the network. The device will routinely be set to an IDLE
state in order to preserve the battery life. In the IDLE
state, the UE will be conducting operations such as cell reselection. But it will also periodically be listening to see whether it is being paged. Any data coming into the 5GC to be sent to the UE requires paging. The network needs to know where that device is.
However, the problem is that the cell reselection that takes place in an IDLE state is done autonomously and the device does not keep the network updated as to which specific cell it’s in. If we need to page that UE, what we don’t want to have to do is page every single gNB in the NG-RAN. So, consequently, the NG-RAN is broken down into tracking areas. What’s crucial in the system is that the UE might be autonomously making cell reselection when it is in the IDLE
mode. The UE will keep the network updated as to which tracking area it is currently in. A tracking area is simply an administrative collection of gNBs and their associated radio coverage, as depicted in the figure.
The 5G Core Access and Mobility Management Function (AMF) in the 5GC keeps track of the tracking area that the subscriber is currently in. So, the subscriber’s UE will be required to update the network any time it moves into a new tracking area. The User Plane Function (UPF) in the 5GC is responsible for the user plane data. If user data comes into the UPF, it will inform the AMF and the AMF will page a specific tracking area instead of paging every gNB in the network, since it already knows which tracking area the UE is in.
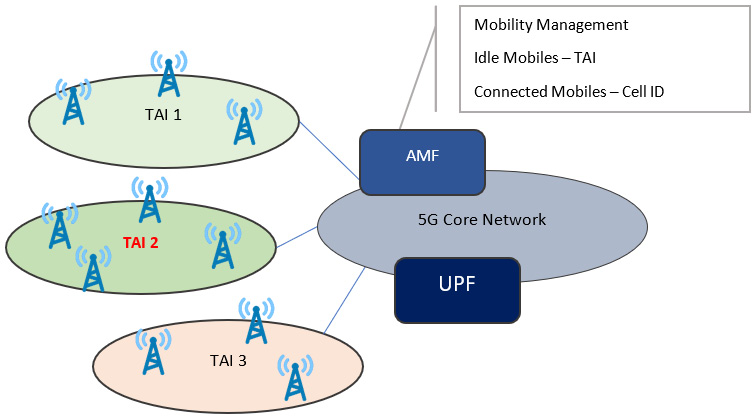
Figure 1.6 – Tracking areas
The tracking areas effectively make paging much more efficient. As soon as the UE connects to the network, the AMF will know its cell ID. But when it is IDLE, it just knows the location of the UE to the granularity of the tracking area. Tracking area planning is all about making the paging more efficient.
5G RAN deployment options
Service providers will be in a transition phase as they move from 4G toward 5G networks. They can’t just switch on the 5G network suddenly. There are some strategy options for service providers in terms of migration. Now notice that in the diagram, there are 4G EPC and 5GCs. The first question is, will the service provider be deploying the 5GC or 5G Radio Access Network first or will they both be deployed in parallel? Either way, there are various connectivity and deployment options available for the service providers to choose from. It is not necessarily the case of one or the other being used; it could be a mixture.
With the NSA approach, there is a 5G gNB, which supports dual connectivity to a 4G eNB, which stands for Evolved Node B. So, the UE will be in communication with both RAN nodes together. It will have 4G radio connectivity to the eNB and 5G radio connectivity to the gNB. With the help of 5G radio connectivity, service providers can provide 5G services to their customers. In this approach, notice that the control connectivity goes back into the 4G EPC since the eNB is the primary RAN device in this architecture. So, this approach provides the benefits of 5G gNB with 5G RAN coverage. Alternatively, 5GC can also be utilized. In this approach, there is a gNB again and that gNB is in communication with a Next-Generation eNB (ng-eNB). The main difference is that the ng-eNB connects to the 5GC. But it’s a similar scenario whereby dual connectivity is used with the gNB as a secondary device and the ng-eNB as a primary device or master device and that control comes from the 5GC down to the ng-eNB. Both these options are NSA. It depends on the service provider as to which approach they want to go for.
The second option is SA, which is a pure 5G deployment. The UE is using the gNB and that gNB is connecting directly to the 5GC.
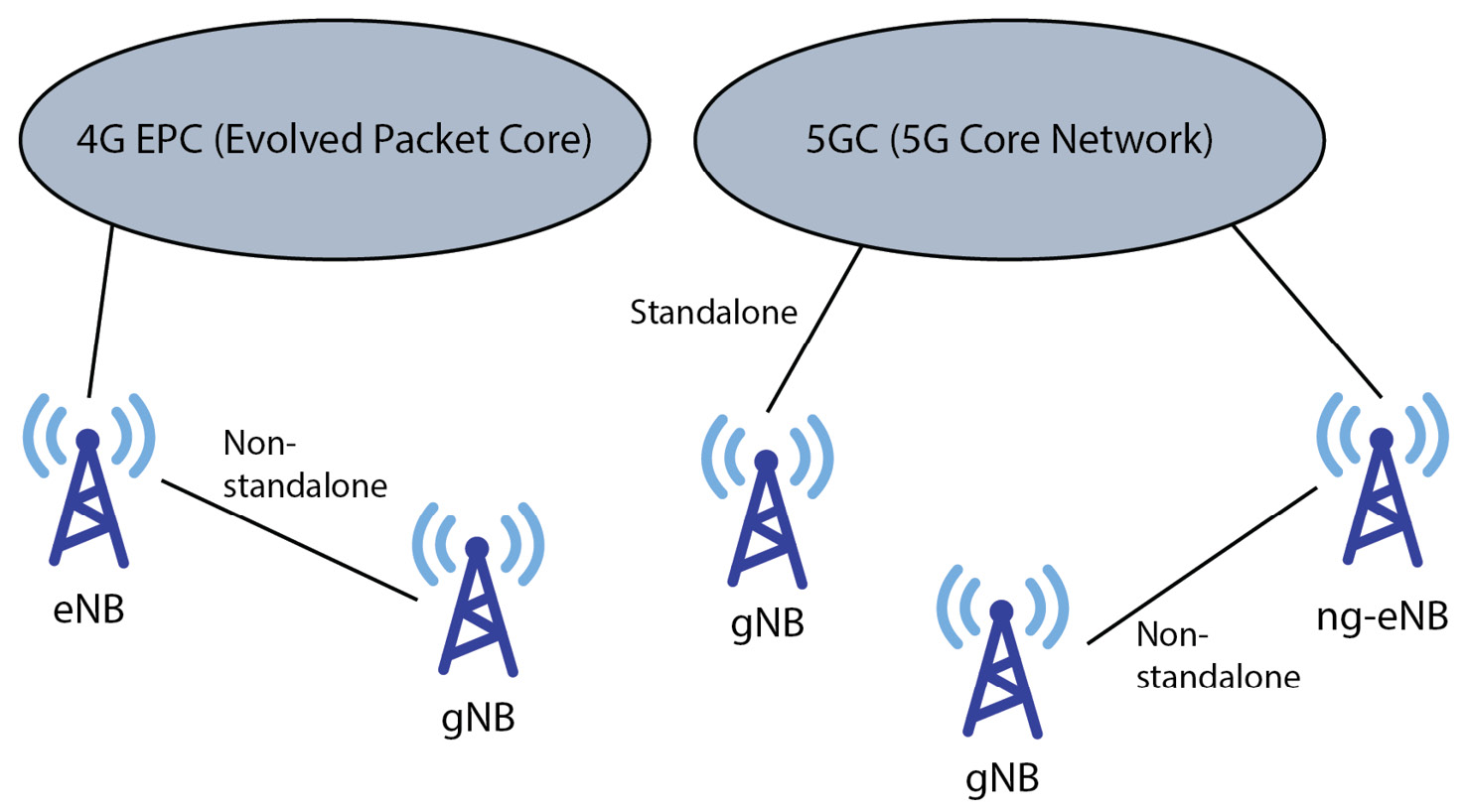
Figure 1.7 – 5G Radio Access Network deployment options
Certainly, the NSA option is more straightforward to implement with a smaller investment. However, in the long term, network architectures and thus network deployments will be based on SA.
NR and NG-RAN features
To meet the requirements of IMT-2020, such as coverage, capacity, and data rates, there are some techniques and technologies that are employed. Let’s look at these in detail.
Dual connectivity
In a typical network deployment, there are UEs in communication with RAN nodes and RAN nodes are connected to the core network through the control plane and user plane. In the context of dual connectivity, the RAN node is the master RAN node. The master RAN node effectively controls any dual connectivity activity that takes place, which includes adding a secondary RAN node. This is where the dual connectivity terminology comes from.
With this approach, a secondary RAN node works in parallel with the master RAN node to improve the effective data rate that the UE can achieve. To accomplish this, there must be a control and user plane connection between the secondary and master nodes. Certainly, the data rate for the subscriber device can be significantly increased by taking this approach.
The terminology used between the master RAN node and the secondary RAN node varies depending on the network architecture and mobile technology. In 5G, for instance, the master and secondary RAN nodes could both be gNBs. Alternatively, the master could be an ng-eNB and the secondary could be a gNB. So, there are several different options available. From the service provider’s perspective, it is up to them how to deploy their dual connectivity solution.
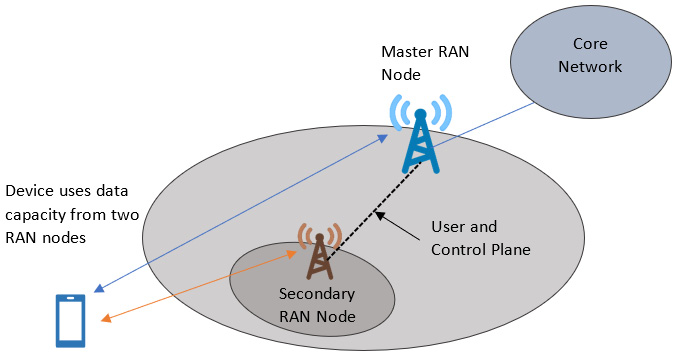
Figure 1.8 – Dual connectivity
Certainly, there are different approaches where a mixture of technologies is used between the master and secondary RAN nodes, as can be found in 5G NSA deployments. Fundamentally, by using these two nodes together, better coverage and improved data rates can be provided for the subscribers. Note that the UE has also got to support dual connectivity. This is particularly important if, for example, the master RAN node is an eNB and the secondary RAN node is a gNB, in which the device is then supporting 4G and 5G radios simultaneously.
Small cells
Small cells are nothing new; they are not a new technology. Small cells have already been in use with previous technologies for several years. In this section, small cells will be examined in the context of dual connectivity in 5G.
5G is set to really benefit from the deployment of small cells. In the following scenario, small cells are providing augmented indoor coverage where in-building penetration of the macro cell, especially in a high-frequency range, might be quite difficult. Consequently, these small cells are deployed within a building to improve indoor coverage and capacity in some cases. However, for 5G, indoor coverage is an important aspect of small cells and indeed outdoor small cells will be routinely deployed.
There are macro-level RAN nodes and small cells with the outdoor small cell deployment approach. These macro-level RAN nodes act as the master RAN nodes, whereas the small cells act as the secondary RAN node within the dual connectivity deployment.
As the UE moves through the network, the blue line shows us the coverage that is experienced from the macro-level RAN nodes. The small cells provide data rate boosts to the UE as it moves through them. Therefore, in a dense urban environment, these small cells, which may only have a range of hundreds of meters, can provide that augmented data rate boost to the network while increasing the overall capacity.
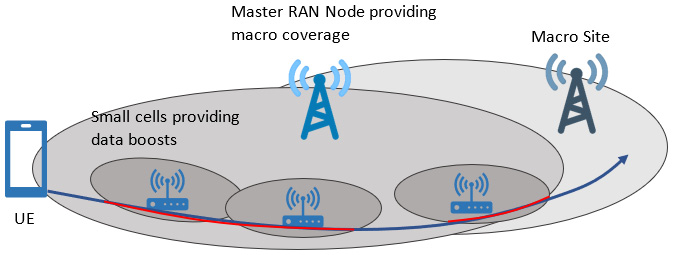
Figure 1.9 – Macro coverage with small cells
These small cells can be set on the top of street poles, street furniture, lampposts, and so on. Small cells are a strong deployment option for service providers to really achieve the data rates expected for 5G.
Increased spectrum
In meeting the target of 100 megabits per second as a mean data rate, or potentially 20 Gbps as a peak data rate, it is essential for the service providers to have access to a more licensed spectrum. Consequently, the licensed spectrum bands that are being considered for 5G operation have been greatly increased.
For 5G deployments, there are several bands in use. They are below 1 GHz, 1-6 GHz, and 6-100 GHz bands. The below 1 GHz and 1-6 GHz bands are not new, and they are used by service providers quite routinely. 6-100 GHz is really the new band. So, let’s explain why this new band is needed.
Below 1 GHz is excellent for building penetration with wide area coverage. The coverage is potentially about tens of kilometers depending on the topography. But the problem with operating below 1 GHz is that there is not that much spectrum available for service providers, that is, there is limited spectrum availability. So, what we need to do is start looking higher up in the radio spectrum.
1-6 GHz provides decent coverage, and there is also good spectrum availability. 6-100 GHz is low range and only provides hundreds of meters of coverage, but there is greater amount of spectrum available. However, it is the key enabler for unlocking the stringent data rate requirements. Service providers will be operating in much bigger bands higher up in the licensed Radio Frequency (RF) spectrum, which is essential for providing those data rates.
As it has been with technologies that have come before and at the same time as 5G, ITU is responsible for standardization and global harmonization of the RF spectrum. At the World Radio Conference in November 2015, they already started to discuss and define some of the operating bands for 5G, and at the World Radio Conference in October 2019, those bands were set in place.
Radio enhancements
5G NR means that the UE needs to be able to support 5G radio, and so too does the gNB. There are many different tweaks that have been employed in NR protocols. However, only two high-level aspects are going to be examined here.
Fundamentally, one of the big changes is the employment of Cyclic Prefix Orthogonal Frequency Division Multiplexing (CP-OFDM) to provide greater deployment flexibility, which adopts variable subcarrier spacing. This helps service providers obtain much more flexibility in terms of what kind of RAN coverage they want to provide. Therefore, CP-OFDM allows them to address whether to deploy a small cell or a very large macro cell while allowing them to be very flexible in terms of the frequency range that they can operate on. Moreover, variable subcarrier spacing allows addressing specific latency requirements.
To address the requirements of the different IMT-2020 use cases, there will be a mixture of different cell types. Some cells might require low latency and high frequency, whereas others might be long-range cells. Therefore, to accommodate those different IMT-2020 requirements, such as latency and coverage, CP-OFDM has been introduced.
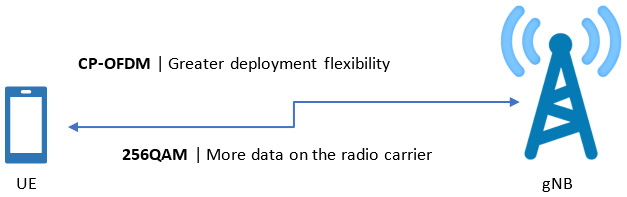
Figure 1.10 – Radio enhancements
The other key area that has been introduced with 5G NR is the use of 256 Quadrature Amplitude Modulation (256QAM), which is already in use with LTE Advanced technology. This is simply a radio modulation technique and effectively allows squeezing even more data onto the radio carrier, hence increasing those data rates as appropriate. However, to utilize 256QAM the UE needs to be in a very good radio coverage environment.
Beam forming
On the left-hand side of Figure 1.11, traditional antenna coverage is represented. Traditionally, the antennas might be sectored so they cover quite a large area. Within that coverage area, there might be fixed wireless access subscribers within the houses, mobile phones, or even fast-moving subscribers. But the idea of traditional antennas is to cover a wide area.
In 5G, Massive MIMO is set to be used. A Massive MIMO antenna is a totally new antenna design that comprises a huge number of radio frequency elements, in effect radio frequency antennas.
One of the key advantages of Massive MIMO antennas is being able to form beams of radio frequency energy. This Massive MIMO array can provide much finer grain coverage. If there is a UE within that beam of coverage, the UE will get better general RAN coverage, which means the technologies such as 256QAM can be used to achieve the optimum data rate.
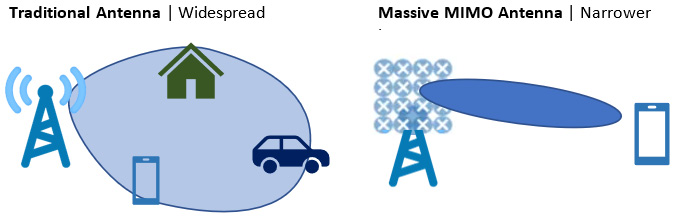
Figure 1.11 – Beam forming with Massive MIMO
It is not only about creating that narrower beam of radio frequency energy; Massive MIMO antennas should be able to do that for numerous subscribers within the cell. So, the beam will constantly be flicking around to service different subscribers, which is called beam steering.
Beam steering
Beam steering is the concept of beams following a UE in the network. If the UE does not move too quickly, it can constantly provide feedback to the antenna so that the antenna can adjust the direction of the beam that it is sending. It is great for slow-moving UEs, while it is more challenging for fast-moving UEs for the beam to keep track of them. Basically, it depends on the speed of the UE whether the beam steering is used or not.
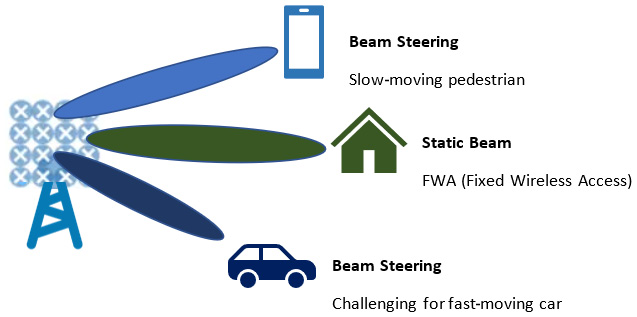
Figure 1.12 – Beam steering with Massive MIMO
Beam forming and beam steering are considered to be critical areas associated with 5G RAN deployments.
Cloud RAN
The final point related to 5G NR and NG-RAN techniques and technologies is the notion of cloud RAN. Essentially, cloud RAN sees the introduction of virtualization technologies to the radio access network. With cloud RAN, each individual gNB has separate compute and processing resources. The idea behind cloud RAN is to take that compute and storage capability and move it to a Centralized Unit (CU). This CU is responsible for conducting the processing and computing activities of all these gNBs. So, the idea here is the compute capabilities are centrally located in a data center and all that remains is down at the cell site are distributed units.
The distributed units are the transmit and receive elements of the gNB. This helps RAN deployment to be simplified and potentially cheaper. The central controlling element fundamentally is sending to the distributed units what they need to transmit. The distributed units are simply responsible for transmitting, receiving, and exchanging that traffic with the CU.
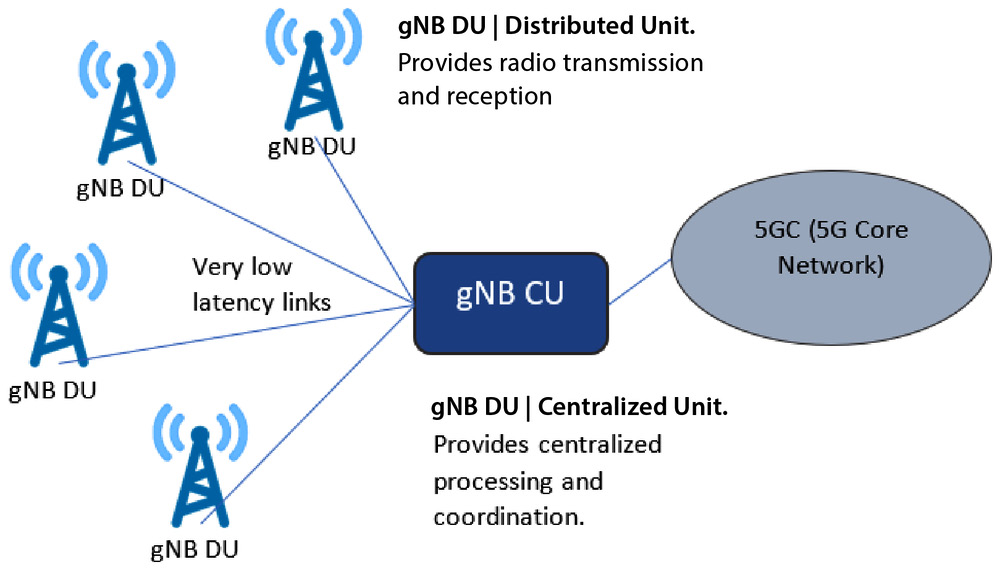
Figure 1.13 – Cloud RAN high-level architecture
The optical transmission links that connect the CU to the distributed unit in this approach are very critical. Fundamentally, these links must meet very low-latency requirements and they can be over a kilometer long. But in summary, here, the CU is simply using virtualization technologies to control several distributed units.
In this section, we analyzed the concepts of NG-RAN architecture, 5G RAN deployment options, and NR and NG-RAN features, which create the basis for the upcoming chapters, where we will examine them in detail.